Fluorescence imaging is a cutting edge technique wherein fluorescent dyes called fluorophores act as labels that expose molecular processes in cells and tissues. With the resolution to peer down to the single cell level, fluorescence imaging could have a dramatic impact on disease prognosis, diagnosis and therapy. However, current techniques only image in the visible portion of the electromagnetic spectrum, where absorption from water and haemoglobin in the blood creates a barrier to probing any deeper than the micrometre level. To break through this barrier, fluorescence imaging techniques need to view cells in the near infrared (NIR) region, ideally in the NIR-II window (1000–1700 nm). But NIR/NIR-II fluorophores have low quantum yields, essentially making them not very bright, and therefore hard to detect. Research published in Nanoscale describes ground-breaking results from an international team led by Imperial College, London, who have used gold nanostars that interact with fluorophores to achieve a 19-fold cellular imaging fluorescence enhancement in the NIR, paving the way for novel high-performance in vitro and in vivo diagnostic platforms.
Tiny particles with big biomedical potential
As the name gives away, nanostars are star-shaped nanoparticles, 1–100 nm in diameter. Formed from various metals, including gold, silver and platinum, they consist of a spherical core surrounded by multiple spikes of varying length, width and sharpness. Gold and silver nanostars are particularly interesting in biology due to their high surface-to-volume ratio, biocompatibility and chemical stability, and their unique optical properties.
These interesting properties have prompted a research team led by Imperial College, London, to investigate gold nanostars for various applications in biology. Alexandra Porter and colleagues, for instance, explored how to synthesise gold nanostars that actively target cancer for use in photothermal therapy in 2017. “If you feed these gold nanostars to triple negative breast cancer cells – one of the most aggressive forms of breast cancer that can’t be treated with hormone therapy – and then excite them with a laser, they become more sensitive to chemotherapeutic drugs, meaning lower doses are needed to treat the cells.”
Gold nanostars enhancing fluorescence
More recently Porter has been involved in a project led by Imperial colleague Fang Xie to use gold nanostars in order to drastically improve fluorescence imaging so that it can be used in detecting diseases such as cancers.
Current clinical methods, including MRI, CT and PET, penetrate deeply into the body to identify tumours. But these methods are only useful when a tumour is already 2–3 cm wide. Meanwhile, fluorescence-based methods naturally have single-cell resolution, making them ideal for detecting much smaller metastases. Yet, currently they can only penetrate micrometres into the body due to the method being restricted to the visible range of the spectrum, limiting their utility. Using gold nanostars that interact with fluorophores, the project aims to enhance the brightness of fluorophores in the NIR region, meaning the technique can probe deeper, anywhere from 1 mm to 1 cm. “In our work, we’re shifting the frequency of imaging,” says Xie. “Gold nanostars amplify the NIR signal so that tissues basically become transparent – and in that way it becomes a form of non-invasive imaging.”


The project rests on one of the most interesting properties of gold nanostars, a phenomenon known as localised surface plasmon resonance (LSPR). LSPR occurs when incident light causes electrons to oscillate resonantly. Nanostars concentrate electron density at the sharp tips of their branches, massively increasing the interaction cross-section of the particle with photons and therefore enhancing the resulting electric field. Moreover, the enhancement and resonance depend on the nanostar’s size and shape, meaning these optical properties can be easily tuned.
In 2018, the team showed that single nanostars interact with fluorophores and indeed enhance fluorescence significantly in solution. This year, they went a step further, for the first time imaging using gold nanostar arrays to show that this tunable enhancement also occurs in cervical cancer HeLa cells – a 19-fold fluorescence enhancement under fluorescence cell imaging conditions.
This achievement opens the door to a number of possibilities. Not least of these is the promise of novel high-performance in vitro and in vivo diagnostic platforms for cancer. “We are hoping this method can pick up cancers at a much earlier stage than current methods,” adds Xie. Moreover, the tunability of fluorescence enhancement suggests it could be wielded in multicolour and multiplexed platforms for a panel of biomarkers, tuning different nanostars to several different fluorophores in the NIR/NIR-II regions to allow diverse subcell populations to be distinguished at the single cell level.
To realise these ambitions, the team is currently examining the toxicology and ultimate fate of their gold nanostars in the body, and trying to understand the mechanisms behind fluorescence enhancement at a fundamental level in order to carefully engineer the chemistry, geometry and size of the particles.

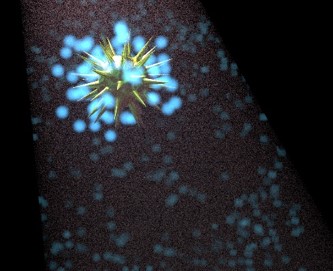
The researchers hope to use equipment provided by the Rosalind Franklin Institute (RFI) in these endeavours. For example, the Institute’s state-of-the-art electron microscopy facilities are ideally suited to mapping the LSPR and how this is affected by the geometry of the nanostars, as Angus Kirkland, RFI Correlated Imaging theme lead attests: “The new RFI instruments will enable high-resolution mapping of the LSPRs which underpin this technology, enabling optimised engineering of the size and morphology of the nanostars”.
At the same time as using the Institute’s facilities to benefit gold nanostar progress, the research has “clear resonance with many of the activities within the RFI Correlated Imaging theme,” says Kirkland. “These nanostars provide greater sensitivity in fluorescence optical microscopy which can be carried out in vivo and subsequently correlated with electron microscopy of frozen samples.”
Porter, who is involved in the Correlated Imaging theme, sees a range of applications that reinforce the RFI mission to translate developments in physical science into biology and ultimately medicine: “With our technique, we could potentially adapt these particles for MRI, CT, etc, and have a capability with much higher spatial resolution,” she says. “And at the lower length scales, we could use fluorescence for confocal microscopy and also tracking for electron microscopy to target specific organelles or membranes.”
To find out more about the Rosalind Franklin Institute’s Correlated Imaging theme, or to discuss potential applications, please contact Theme Lead Professor Angus Kirkland.
References
Theodorou, I G et al. - Fluorescence enhancement from single gold nanostars: towards ultra-bright emission in the first and second near-infrared biological windows. - Nanoscale 10, 15854 (2018)
Theodorou, I G et al. - Towards multiplexed near-infrared cellular imaging using gold nanostar arrays with tunable fluorescence enhancement. - Nanoscale 11, 2079 (2019)
Yuin Ong, Z et al - Multibranched Gold Nanoparticles with Intrinsic LAT‑1 Targeting Capabilities for Selective Photothermal Therapy of Breast Cancer - ACS Appl. Mater. Interfaces 9, 39259–70 (2017)